Discovery to Deployment: The CRISPR Journey From Lab to Life-Changing Therapy
- Nick Inboden
- Jun 6, 2024
- 7 min read
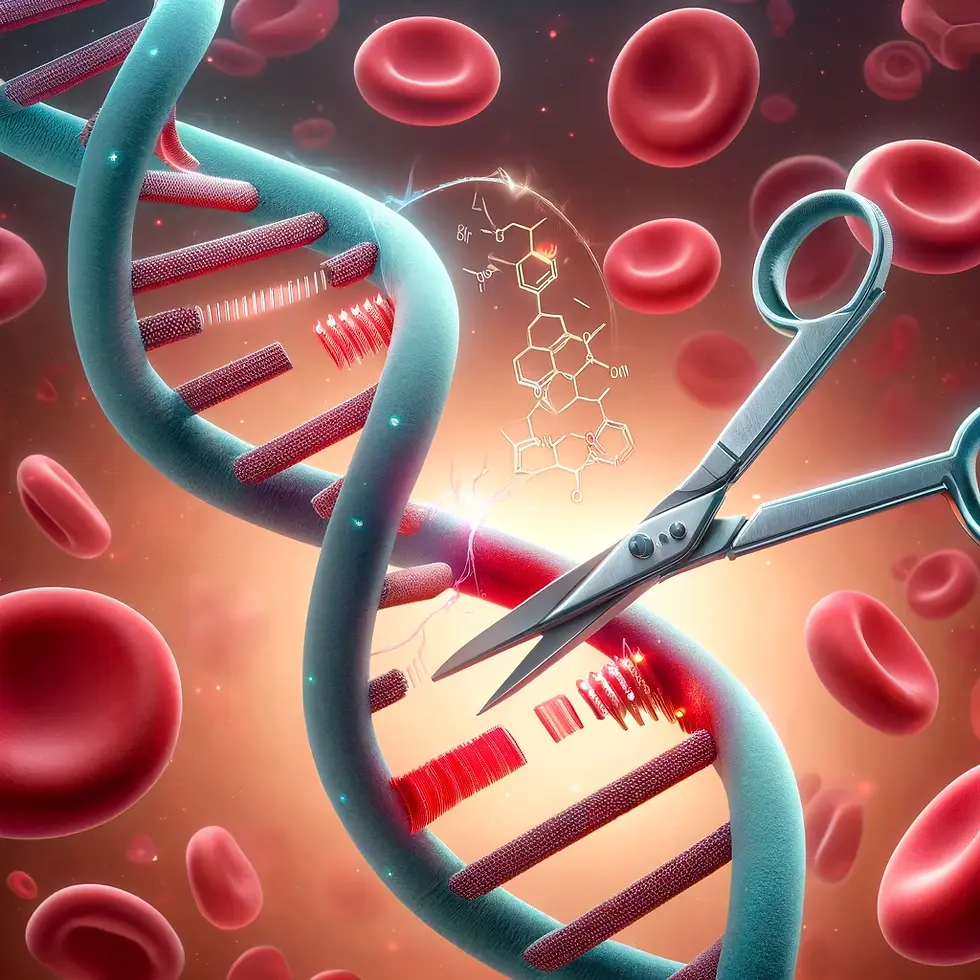
CRISPR technology has emerged as a revolutionary tool in science and medicine, capable of precisely altering DNA. With demonstrated applications in clinical settings, CRISPR has been a significant topic of discussion in biotech and medical circles for years, even sparking ethical debates amongst members of the general public. To truly appreciate its impact, it’s essential to understand how this gene editing tool works and why it has gained so much traction in recent years.
Understanding CRISPR
CRISPR (Clustered Regularly Interspaced Short Palindromic Repeats), discovered in 1987, is a natural system found in bacteria. Bacteria utilize CRISPR to protect themselves from viruses by recording the genetic information of previous viral invaders and using that information to recognize and destroy subsequent viruses. Scientists have figured out how to use this system to edit genes by changing the DNA sequences in living organisms.
CRISPR works like a pair of tiny scissors that can cut DNA at specific places. CRISPR uses a special protein called Cas9 and a guide RNA that tells Cas9 where to cut. Cas9 is simply an endonuclease, a type of enzyme that makes a cut in a DNA or RNA sequence. Cas9 has gained notoriety and is used because of its high specificity. The guide RNA is designed to match 20 nucleotides in the DNA sequence, ensuring that Cas9 cuts at the exact right spot. Since there are 4 different nucleotides (adenine, thymine, cytosine, and guanine), a 20-nucleotide sequence can have 1.1 trillion possible sequences (420). Given that the human genome is composed of about 3 billion base pairs, the likelihood of the guide RNA sequence making an incorrect cut is theoretically 0. Thus, Cas9 can be directed to cut and edit DNA with high specificity and accuracy. This high level of specificity is like having a key that fits perfectly into a lock with 20 unique pins. If even one pin is out of place, the key won't work. Similarly, the guide RNA must match the DNA sequence exactly for the cut to be made. This precision allows scientists to make targeted changes to DNA without affecting other parts of the genome.
Gene editing is like editing a document, but instead of words, DNA is edited. The CRISPR/Cas9 system makes a cut in DNA at a specific spot. The cell then repairs the cut, and scientists can add, remove, or change pieces of DNA during this repair process. This ability to make precise changes has revolutionized genetic research and medicine.
CASGEVY (Exagamglogene Autotemcel or Exa-cel) is a groundbreaking gene therapy designed to treat sickle cell disease using CRISPR/Cas9 technology. After positive clinical trial results, CASGEVY was approved by the FDA on December 8, 2023. The treatment protocol using CASGEVY is detailed:
Patient Preparation / Collection of Blood Stem Cells
Blood Stem Cells must be extracted from the bone marrow into the bloodstream to facilitate collection, a process initiated by administering a medication and often referred to as mobilization. The patient is then connected via IV to a machine that separates the Blood Stem Cells and returns the rest of the blood back to the patient.
Genetic Modification
The CRISPR/Cas9 complex is introduced into the patient's Blood Stem Cells. The guide RNA directs Cas9 to the specific DNA sequence, where it makes a cut in the DNA. A process referred to as electroporation, which momentarily and reversibly breaks down the cell membrane, is used to help transport genetic information into the Blood Stem Cells. The DNA is modified to promote the production of fetal hemoglobin, a healthy form of hemoglobin that prevents sickling and carries oxygen very well.
Hemoglobin is the molecule in red blood cells responsible for carrying and delivering oxygen around the body. There are multiple forms of Hemoglobin that can exist, with fetal hemoglobin being the most powerful due to the fast growing nature of babies.
Cell Expansion
The genetically modified Blood Stem Cells are cultured and multiplied in a laboratory to produce a sufficient quantity for transplantation. A larger number of cells increases the likelihood of a successful transplant.
Pre-Transplant Conditioning
The patient undergoes a conditioning regimen, typically involving chemotherapy, to prepare their body to receive the edited Blood Stem Cells.
Purpose of Chemotherapy:
Creating Space: Chemotherapy helps to create space in the bone marrow by eliminating existing Blood Stem Cells, making room for the transplanted, modified Blood Stem Cells to engraft, or replace the original Blood Stem Cells.
Stem Cell Transplantation
After the gene editing process, the modified Blood Stem Cells are infused back into the patient’s bloodstream via IV. This procedure is similar to receiving a blood transfusion and usually takes a few hours. Once in the bloodstream, these genetically modified Blood Stem Cells travel to the bone marrow. In the bone marrow, they settle and start producing healthy red blood cells. This process of settling and producing healthy red blood cells is referred to as grafting, and is critical for the success of the treatment; it ensures the newly modified Blood Stem Cells can replace the defective ones and begin to function normally.
While the process for treatment using CASGEVY is long and somewhat intense, the effectiveness of the treatment can not be understated. During clinical trials, 93.5% of patients achieved 100% freedom from blockages in the bloodstream due to sickling of red blood cells observed over a period of 12 months. Of all patients receiving the treatment, there were no cases of graft rejection or failure, a true testament to the effectiveness of the gene editing therapy.
Non-Medical uses for CRISPR
When individuals think of editing genes and DNA, their minds often gravitate towards medical advances with potential to cure seemingly “incurable” diseases. While a large portion of research regarding gene editing and CRISPR technology is aimed toward the medical field, applications for gene editing extend far outside the walls of hospitals and labs. Plenty of research is being done to improve operational efficiency for a wide variety of industries, one of the most notable being agriculture.
In the agricultural industry, CRISPR is used to improve crops by enhancing resistance to diseases, improving yield, and increasing resilience to changes in climate. Increasing nutritional value of crops is a topic at the forefront of CRISPR applications in regard to agriculture. For example, the genetic mechanisms responsible for vitamin production in crops can be modified to increase vitamin content, thereby boosting nutrition levels without increasing the quantity of food needed by a consumer to obtain sufficient levels of the nutrient. Since these changes are genetic, improved nutritional traits are passed down to future crop generations simply through their seeds. This technology has significant potential to address issues like world hunger and malnutrition in developing nations. Farmers in developing regions would not need to alter farming methods or invest in expensive new technology to increase the nutritional value of their crops; they would only need seeds produced by genetically modified parent crops.
The Ethical Implications of Gene Editing in Humans
Editing human genes raises important ethical questions. The ability to change human DNA brings up concerns about long-term effects and whether it's morally right to make permanent changes to the human genome.
Public opinion on gene editing varies and has been subject to widespread debate. Some people support the potential to cure diseases, while others worry about unknown risks and ethical issues. Due to the variety of applications of CRISPR/Cas9 technologies, a large portion of the population falls in a gray zone where certain applications, like that of curing diseases, should be permitted, but the application of gene editing technology to make a child taller or more athletic (designer babies) should not.
A famous controversy involving gene editing involved He Jiankui, a scientist listed on Time’s 100 most influential people of 2019, when He edited the genes of twin human embryos which later resulted in the birth of 2 girls named Lulu and Nana. Announced to the general public in 2018, He’s experiment aimed to make the twins resistant to HIV; needless to say, the world was shocked. Leading scientists and researchers criticized the experiment as premature, unethical, and dangerous, calling for a moratorium on human germline editing. Ethicists emphasized the lack of informed consent and the potential for unintended consequences, arguing that such experiments should not proceed without broad societal consensus and robust regulatory frameworks. The CRISPR/Cas9 technology was still relatively new and unproven for use in human embryos, leading to concerns about off-target effects (unintended genetic changes) and the long-term health implications for Lulu and Nana. Editing the genes of embryos results in changes that are heritable, meaning they can be passed to future generations, which raises profound questions about the potential impact on the human gene pool. Additionally, the experiment violated international norms and guidelines for ethical research, including those of the World Health Organization and other scientific bodies. The Chinese government condemned the experiment and launched an investigation. He Jiankui was ultimately found to have violated national regulations and was sentenced to three years in prison. In 2022, He announced he was starting a new lab focused on researching and developing a cure for Duchenne Muscular Dystrophy, a disease that causes progressive degeneration of muscle fiber. CRISPR technology is being used in his new research, but not to edit human embryos. The application of gene editing technology in He’s new lab is more akin to that of CASGEVY.
He’s human embryo experiment underscored the need for stronger international regulations and oversight of gene-editing technologies, leading many countries to review and tighten their regulations on human genome editing. It also intensified ethical debates about the use of CRISPR technology in humans, particularly regarding germline editing, referring to descendants of modified organisms, and the potential long-term effects on humanity. The affair highlighted the importance of scientific responsibility, transparency, and adherence to ethical standards in research, emphasizing the need for ongoing dialogue between scientists, ethicists, policymakers, and the public.
Immense capabilities demand careful stewardship
CRISPR technology has revolutionized gene editing with its ability to make precise DNA modifications. Originating from a natural bacterial defense system, CRISPR/Cas9 acts like molecular scissors, enabling targeted gene alterations. This technology has vast applications in medicine and agriculture, from treating genetic disorders to enhancing crop nutrition. However, ethical concerns, particularly regarding human germline editing, highlight the need for continued dialogue and creation of regulations for responsible use. CRISPR's potential is immense, but it must be harnessed and deployed with care and consideration.
At BioBeacon, we value community insight and would love to hear your thoughts! Join the discussion by leaving a comment below. Have questions or insights to share? Feel free to reach out and get in touch with us. Your engagement is invaluable, and together we can explore the future of biotechnology and medicine. Don't forget to share this post with your network and keep the conversation going!
Kommentare